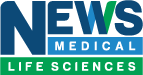
Scientists print 3D structure that mimics the air sacs in the lungs
Scientists have overcome a major obstacle in the development of 3D printed tissue that can be used to replaced damaged or diseased organs. Using breakthrough techniques, the scientist’s 3D printed a structure resembling an alveolar air sac – the structures that carry out gas exchange in the lungs.
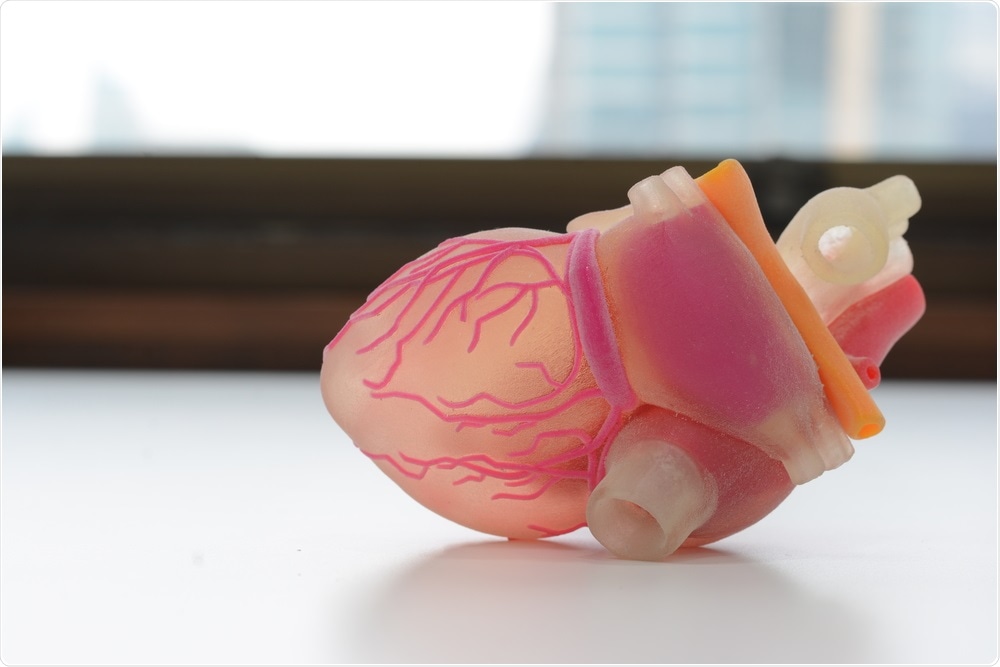
In the current experiment, bioengineers pioneered the use of food dyes as photoabsorbers to use in a process known as novel photopatterning SLATE (stereolithography apparatus for tissue engineering) technology. This was used to create functional complex vascular architectures with hydrogels, via 3D bioprinting.
Previous bioprinting techniques have been hindered by their inability to mimic the complex vascular networks seen in the human body.
Many of these networks intertwine with, yet are distinct from, the anatomical arrangement of the major organs themselves. For instance, blood vessels in the biliary system of the liver closely interact with the liver tissue but do not actually form a part of it.
Biochemically and physically, however, blood vessels must be in close proximity to the organ in order for it to function.
Addressing ‘the challenge of multi-vascularization’
The proof-of-concept model, which was featured on the cover of Science magazine this week, is being described as a visual and functional simulation of the complex networks of blood vessels and air passages that characterize the human lung’s alveolar sac anatomy.
It is hoped that the new technology will empower other scientists to produce similarly complex networks that duplicate the natural passages for air, lymph, blood and other body fluids.
SLATE is a novel open-source hydrogel-based 3D bioprinting method that works perfectly to build up a complex monolithic soft hydrogel with the required topology.
It all begins with a pre-hydrogel liquid that solidifies on exposure to blue light. 2D slices of the required structure are displayed in sequence by a blue light beam shining from below, from a digital processor.
This causes the hydrogel solution to solidify in extremely fine layers, one at a time, to follow the extremely complex design of the vascular architecture. This creates a biocompatible water-based gel model within minutes.
Using common food dyes was a breakthrough
The breakthrough that enabled the duplication of vascular architecture was the addition of cell-compatible photoabsorbers to the solution, in the form of commonly used food dyes that absorb blue light. These include anthocyanin, curcumin and tartrazine.
The bioprinted vascular tissue passed tests of its ability to withstand normal blood pressure variations and pulsatile flow, as well as simulated breathing movements that mimic human breath frequencies and pressures via air inflow and outflow.
It also allows oxygen uptake by red cells as they pass through a vascular network around the air sac, similar to lung alveolar phenomena.
The breakthrough research provides hope for millions of patients on the transplant list around the world, as we move one step closer to printing ready-made organs for patients.
Printing tissues that ‘breathe like the healthy tissues in our bodies’
The researchers then focused on creating a similar model for the liver.
The liver is highly complex with over 500 functions, second only to the brain in the breadth and magnitude of its workload. Currently, no therapeutic protocol or medical device can successfully replicate the function of the liver, so patients with liver damage must wait for an organ to become available for transplant.
As part of the current study, the team printed 3D vascular tissue mimicking the liver vasculature and then added primary liver cells in separate compartments. These therapeutic implants were then transferred into mice with chronic liver damage.
Subsequent testing revealed that the liver cells stayed alive and functional through this process. This could help accelerate the development of liver implants as well.
Other possibilities include bioprinting of finer features within blood vessels, such as one-way bicuspid valves. These valves ensure unidirectional blood flow towards the heart in the absence of a separate pump and are found in the heart and lymph vessels.
The research was led by bioengineers Miller and Kelly Stevens and included researchers from Rice University, University of Washington, and Rowan University, and Nervous System, a design firm.
While some of the research is being turned into a commercial product by Volumetric, a start-up in Houston, all the source files are available for free. This includes a complete set of 3D printable files for the SLATE and for each of the bioinks (the hydrogels) used in this study.
Source:
Grigoryan B., et al. (2019). Multivascular networks and functional intravascular topologies within biocompatible hydrogels. Science. science.sciencemag.org/content/364/6439/458
No comments:
Post a Comment